Kraft
(sulfate) process
Sulfite
process
Basis
weight
Grammage
Chapter 8. Pulp and Paper
|
Pulp is the fibrous mass that results when a pulping process
ruptures the bonds in the wood structure that hold the woody cells
together. Pulping is done mechanically, thermomechanically, chemically,
or with combinations of these treatments. Commercial processes are
generally classified as mechanical, chemical, or semichemical-the
latter being various combinationsof chemical and mechanical. Appendix
2, Section G, shows that 82% of 1986 production was chemical pulping,
of which 91% is produced by the kraft (sulfate) process. Semichemical
pulping accounts for about 7% of production, and various mechanical
pulping processes account for the remainder.
Pulp statistics are usually reported in units of weight. The most
common are
short ton = 2,000 lb = 907 kg
metric tonne = 1,000 kg = 2,204 lb.
Pulp is generally reported as an air-dried product that is assumed
to be 10% water and 90% oven-dry pulp. The actual condition of a
shipment may vary somewhat from this definition. Pulp is commonly
sold in bales (32 x 32 x 15 inches) which weigh about 500 pounds.
Pulp Yield
Recovery from pulping wood is commonly expressed as
the percentage, by oven-dry weight, of pulp obtained from the original
wood weight. A recovery value of 45% means that for every 100 oven-dry
pounds of wood processed, 45 oven-dry pounds of pulp is produced.
When expressed in this manner, pulp yield is mainly a function of
the pulping process. There is some difference between hardwoods
and softwoods when pulped by chemical processes, due to the difference
in chemical makeup between these groups. Table
8-1provides a summary of common pulping processes and yields.
Section G of Appendix 2 shows percentage use of hardwood and softwood
species.
Mechanical Pulping
The earliest and one of the most common mechanical pulping
methods is the groundwood process, in which a roundwood
bolt is pressed lengthwise against a rough, revolving grinding
stone. The wood fibers are torn out of the wood, abraded, and removed
from the stone surface with water. A different process, called refiner
mechan- ical pulp (RMP) utilizes chips, which are
shredded into fibers between large rotating disks of a device called
a refiner. The basic RMP process has evolved to employ thermal
and/or chemical presoftening of the chips, which reduces energy use
and modifies resultant pulp properties; this is typically termed thermomechanical
pulp (TMP). Mechanical pulping has the advantage of converting
up to 95% of the dry weight of the wood input into pulp, but the mechanical
action requires a large energy input. The pulp forms a highly opaque
paper with good printing properties, but the paper is relatively weak
and discolors easily with exposure to light. Newsprint is a major
product of mechanical pulp. Mechanical pulps are generally produced
from long-fibered softwood (conifer) species. The smaller, thinner
fibers from hardwoods are more severely damaged by the mechanical
action, hence yield a weaker paper. However, some hardwoods, such
as poplar, which produces very bright pulp, are mechanically pulped
and blended with softwood mechanical pulps to improve optical properties.
Chemical Pulping
In chemical pulping, the fibers in wood are separated
by dissolving away the lignin com-ponent, leaving behind a fiber
that retains most of its cellulose and some hemicellulose. Yields
of chemical processes are on the order of 40 to 50% of the dry weight
of the original wood input.
Kraft (Sulfate) Process. Because of
advantages in chemical recovery and pulp strength, the kraft process
dominates the industry. It represents 91% of chemical pulping and
75% of all pulp (Appendix 2, Section G). It evolved from an earlier
soda process (using only sodium hydroxide as the active chem-ical)
and adds sodium sulfide to the cooking chemical formulation. This
is the process associated with the foul odor problem in the environment.
A number of pulp grades are commonly produced, and the yield depends
on the grade of product. Unbleached pulp grades, characterized by
a dark brown color, are generally used for packaging products and
are cooked to a higher yield and retain more of the original lignin.
Bleached pulp grades are made into white papers. Figure 8-1 shows
a pulping curve and relationship between yield, kappa number, and
corresponding product. Nearly half of the kraft production is in
bleached grades, which have the lowest yields. In Figure 8-1, kappa
number refers to the result of a test of the amount of residual
lignin in the pulp-lower kappa meaning less lignin. Effective alkali
and sulfidity refer to conditions of certain key chemical aspects
of the process (for details see Smook 1992). Curves such as shown
in Figure 8-1 also differ with wood species.
Figure 8-1. Kraft pulp yield
vs. kappa and effective alkali charge. Source: Smook (1992). |
|
|
Sulfite
Process. This process uses different chemicals to attack
and remove lignin. Compared to kraft pulps, sulfite pulps are
brighter and bleach more easily, but are weaker. Sulfite pulps
are produced in several grades but bleached grades dominate
production. Yields are generally in the range of 40 to 50%,
but tend toward the lower end of this range in bleached grades.
Compared to the kraft process, this operation has the disadvantage
of being more sensitive to species characteristics. The sulfite
process is usually intolerant of resinous softwoods, tannin-containing
hardwoods, and any furnish containing bark. |
Hybrid Pulping
Methods
A number of processes are hybrids of chemical and mechanical
methods and have intermediate yields. Generally, chips are partly
softened or digested with chemicals and then are mechanically converted
to fiber, usually in disk refiners. Chemimechanical pulping,
typically used on hardwoods, softens chips prior to usual mechanical
action and has yields in the 80 to 90% range. Semichemical
pulping involves greater cooking and delignification prior to mechanical
refining; yields are somewhat lower, depending on the degree of cooking.
Dissolving Pulp
Dissolving pulps are specialty pulps used for chemical conversion
into products such as rayon, cellophane, and cellulose acetate. These
pulps can be made by either a modified kraft (prehydrolysis) or sulfite
process in which the aim is to obtain a pulp of pure cellulose. Since
essentially all lignin and hemicellulose are removed, dissolving pulps
have the lowest yields, on the order of 35%.
Estimating Wood Required
per Ton of Pulp
It is also common to express pulp yield as air-dry
or oven-dry tons of pulp per cord or other unit of wood volume. Alternatively,
the reciprocal of these measures indicates the number of cords or
other wood unit required per ton of pulp. In addition to the factors
discussed above, these measures are affected by wood density. Yield
is generally higher (less wood is required) for denser species. To
estimate wood required per ton of pulp, the following information
is needed: (1) moisture content of pulp, (2) pulp yield, and (3) specific
gravity of the species used as raw material. There are several variations
in calculating wood requirements, due to the different measures of
pulp (short ton, metric tonne) and different measures for the wood
raw material (cubic foot, cubic meter, bone-dry unit). A bone-dry
unit (BDU) represents 2,400 pounds of chips or residues. See Chapter
7 for further discussion of chip and residue measures and conversions.
Basic formulas are:
Formula 1. MCw = [(WP - DP) / WP]
* 100
MCw = pulp moisture
content on total
weight basis (%)
WP = pulp weight including moisture
DP = oven-dry weight of pulp
Formula 2. Y = (DP / DW) * 100
Y = pulp yield (%)
DW = oven-dry weight, wood raw
material
Formula 3. DW = V * SGg
* F
V = green volume of wood raw
material
SGg = wood specific
gravity (see Table 1-1)
F = density of water = 62.4
lb/ft3 or
1,000 kg/m3
In Examples 1 through 7 the pulp moisture content is assumed
to be 10%. These examples illustrate both the information needed
and computational steps involved in developing the common conversion
factors of interest. The reader can substitute yields for other
processes, specific gravities for other species, and other pulp
moisture contents. Appendix 2, Section G, presents overall average
values of wood consumption in manufacturing pulp in 1986. It should
also be noted that about 31% of the solid pulpwood used is actually
in the form of residue by-products from other wood industries (Haynes
1990).
How many
cubic feet of solid western hemlock wood are needed to produce one
short ton (2,000 lb) of oven-dry bleached kraft pulp (kappa 30)?
Formula 1 gives
10 = [(2,000 lb - DP) / 2,000 lb] * 100
solving: DP = 1,800 lb of oven-dry fiber per ton of pulp.
Formula 2 gives
45 = (1,800 lb / DW) * 100
solving: DW = 4,000 lb oven-dry wood per ton of pulp.
Here the
yield of bleached kraft pulp is assumed to be 45% (Figure 8-1).
Formula 3 gives
4,000 lb = V * 0.42 * 62.4 lb/ft3
solving: V = 153 cubic feet of green wood per ton of pulp.
Here 0.42 is the specific gravity of western hemlock
(Table 1-1).
How many
cubic meters of solid western hemlock wood are needed to produce
one tonne (1,000 kg) of oven-dry bleached kraft pulp (kappa 30)?
Formula 1 gives 10 = [(1,000 kg - DP) / 1,000 kg] * 100
solving: DP = 900 kg of oven-dry fiber per tonne of pulp.
Formula 2 gives 45 = (900 kg / DW) * 100
solving: DW = 2,000 kg oven-dry wood per tonne of pulp.
Here the yield of bleached kraft pulp is assumed to be 45% (Figure
8-1).
Formula 3 gives 2,000 kg = V * 0.42 * 1,000
kg/m3
solving: V = 4.76 cubic meters of green wood per tonne of
pulp.
Here 0.45 is the specific gravity of western hemlock (Table 1-1).
How many
cords of western hemlock wood are needed to produce one short ton
of oven-dry bleached kraft pulp?
Example 1 found that 153 cubic feetof solid green wood are needed
per ton of bleached Douglas-fir kraft pulp. Assuming a cord contains
80 cubic feet of solid wood (Chapter 3), this is equivalent to 1.91
cords/ton.
How many
cubic feet of gravity-packed western hemlock chips are needed to
produce one short ton (2,000 lb) of oven-dry bleached kraft pulp?
To answer this question, one must know the relationship between wood
volume before and after chipping. This depends on chip geometry, degree
of settling and compaction, and so forth, which are discussed in Chapter
7. In this example it is assumed that chips occupy 2.5 times the original
solid wood volume (see Table 7-1). Example 1 found that 153 cubic
feet of solid western hemlock wood were needed to produce a ton of
pulp. The equivalent amount of chips is 2.5 times greater, or 382.5
cubic feet.
How many
"units" of western hemlock chips are needed to produce
a ton of oven-dry bleached kraft pulp?
A "unit" typically refers to a 200 cubic foot container.
Example 4 found that a ton of bleached kraft pulp requires 382.5 cubic
feet of western hemlock chips. Division by 200 reveals that this is
equivalent to 1.91 units.
How many
bone-dry units (BDU) of chips are needed to produce an oven-dry
ton of pulp?
Example 1 found that 4,000 pounds of oven-dry wood are needed per
ton of pulp. Since one BDU equals 2,400 pounds of oven-dry wood, the
answer is
(4,000
lb/ton pulp) / (2,400 lb/BDU) = 1.67 BDU/ton pulp.
How many
bone-dry units (BDU) are needed to produce an oven-dry tonne of
pulp?
Multiply the result from Example 6 by
(2,204 lb/tonne) / (2,000 lb/ton) = 1.102 ton/tonne.
Hence, 1.67 BDU/ton * 1.102 ton/tonne = 1.84
BDU/tonne.
Paper and Paperboard
When paper and paperboard are produced, converted, and
sold, several measurements are important. This section briefly discusses
them and provides some illustrative data. Industry statistics are
usually reported on a weight basis, and the standard weight conversions
found in Appendix 1 can be used.
Basis Weight and Grammage
When paper and paperboard are produced and converted, two
key characteristics are weight and area. Production is generally reported
in tons, and products are sold on either a weight or an area basis
depending on the needs of the customer.
Basis Weight. The common measure
in the United States, basis weight,is"the weight, in pounds,
of a predetermined number of sheets having a specific size" (Scott
1989). The predetermined number of sheets is the ream. Generally
500 sheets form a ream, but a ream contains 480 sheets for some products.
Since various producers and converters need different size sheets,
a variety of basic sizes have evolved over time. Table 8-2 indicates
basic size and basis weights for several grades (500 sheet ream).
For example, cover papers have basis weights of 40, 50, 60, 65, 70,
80, 100, and 130; all are based on a 500 sheet ream of 20 by 26 inch
sheets. Unfortunately, these basic sizes have no common relationship;
hence, conversions among them require knowledge of actual sheet size
and number of sheets involved. Essentially, the calculations require
prorating the actual size and number of sheets to the standard basis
size for the grade and to the number of sheets in a ream. Saltman
(1991) provides a number of illustrative practical examples. To further
complicate matters, the U.S. Government Printing Office uses a unit
of 100 sheets, and basis weight for paperboards is usually given as
pounds per 1,000 square feet. The U.S. system is very cumbersome and
can lead to confusion and misunderstanding. Grammage, as defined below,
is gaining popularity, since it is simpler and is widely used internationally.
Grammage. In the SI or metric system,
basis weight is called grammage, which is the weight in grams
per square meter (Scott 1989). This definition eliminates confusion
caused by different standard sizes and different ream counts. Table
8-2 shows how basis weights of various grades relate when expressed
in grammage. In the U.S. system, book and cover papers both have a
basis weight of 50 within the range for each of these grades. Table
8-2 shows that the grammages are 74 and 135, respectively. This difference
was masked by the U.S. practice of measuring basis weights on 25 by
38 inch and 20 by 26 inch areas, respectively. Table 8-3 provides
factors for converting basis weights.
Thickness (Caliper)
Thickness is an important determinant of stiffness. In addition,
paper thickness determines overall thickness of finished products
such as books, and hence the space they will occupy. In the United
States, thickness is in inches, with the terms point and mil
used to refer to thousandths of an inch. In the SI or metric system,
thickness is in micrometers. Table 8-4 presents comparable thickness
measures for several paper grades.
Density and Bulk
Two additional properties of interest are density and bulk.
Apparent density, in grams per cubic centimeter, is obtained by dividing
grammage by thickness. Density in grams per cubic centimeter (g/cc)
can be converted to pounds per cubic foot (lb/ft3)bymultiplying
by 62.4. Density influences most physical, mechanical, and other properties.
Bulk is the reciprocal of density. It measures the volume in cubic
centimeters (cc) occupied by one gram of paper or the volume in cubic
feet occupied by one pound of paper. See Example 8.
Tables 8-2 and 8-4 indicate that index bristol, with grammage of
253 g/m2 (basis weight 140 lb), has a thickness of 0.25
mm (0.0098 inch). Density, g/cc = 253 g/m2 /(1,000
* 0.25 mm) = 1.012 g/cc.(The number 1,000 results from
1 m2 = 10,000 cm2 and 1 cm = 10 mm.) Density,
lb/ft3 = 1.012 62.4 = 63.2 lb/ft3
or
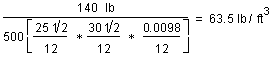
Bulk, cc/g = 1/1.012 = 0.988 cc space per gram.
Bulk, ft3/lb = 1/63.2 = 0.016 ft3 space
per pound.
Table 8-2. Examples of basis
weight and grammage for several grades of paper, based on a 500
sheet ream
(basic sheet size in parentheses).
Basis weight (lb)
|
Bond, ledger,
mimeo, writing (17 x 22) |
Cover(20 x 26) |
Postcard,
wedding bristol
(22-1/2 x 28-1/2) |
Index bristol
(25-1/2 x 30-1/2) |
Glassine, news,
tissues, wrapping
(24 x 36) |
Book, bible,
offset, blotting
(25 x 38) |
Grammage
(g/m2) |
|
x |
|
|
|
x |
20 |
30 |
9 |
|
|
|
x |
x |
34 |
x |
|
|
|
x |
24 |
36 |
11 |
|
|
|
x |
x |
41 |
x |
|
|
|
x |
30 |
44 |
|
|
|
|
|
|
|
13 |
|
|
|
30 |
33 |
49 |
x |
|
|
|
x |
35 |
52 |
15 |
|
|
|
x |
x |
56 |
x |
|
|
|
x |
40 |
59 |
16 |
|
|
|
x |
x |
60 |
|
|
|
|
|
|
|
x |
|
|
|
40 |
x |
65 |
x |
|
|
|
x |
45 |
67 |
x |
|
|
|
x |
50 |
74 |
20 |
|
|
|
x |
x |
75 |
x |
|
|
|
50 |
x |
81 |
|
|
|
|
|
|
|
x |
|
|
|
x |
60 |
89 |
24 |
|
|
|
x |
x |
90 |
x |
|
|
|
60 |
x |
98 |
x |
|
|
|
x |
70 |
104 |
28 |
|
|
|
x |
x |
105 |
|
|
|
|
|
|
|
x |
40 |
|
|
x |
x |
108 |
x |
x |
|
|
70 |
x |
114 |
x |
x |
|
|
x |
80 |
118 |
32 |
x |
|
|
x |
x |
120 |
x |
x |
|
|
80 |
x |
130 |
|
|
|
|
|
|
|
x |
x |
x |
|
x |
90 |
133 |
36 |
50 |
x |
|
x |
x |
135 |
x |
x |
x |
|
90 |
x |
146 |
x |
x |
67 |
|
x |
x |
147 |
x |
x |
x |
|
x |
100 |
148 |
|
|
|
|
|
|
|
40 |
x |
x |
x |
x |
x |
150 |
x |
60 |
x |
x |
x |
x |
162 |
x |
x |
x |
90 |
100 |
x |
163 |
x |
x |
80 |
x |
x |
x |
175 |
x |
65 |
x |
x |
x |
x |
176 |
|
|
|
|
|
|
|
|
x |
x |
x |
x |
120 |
178 |
|
70 |
x |
x |
x |
x |
189 |
|
x |
90 |
x |
x |
x |
197 |
|
x |
x |
110 |
x |
x |
199 |
|
x |
x |
x |
125 |
x |
203 |
|
|
|
|
|
|
|
|
80 |
x |
x |
x |
|
216 |
|
x |
x |
x |
x |
|
218 |
|
x |
100 |
x |
x |
|
219 |
|
x |
x |
x |
150 |
|
244 |
|
x |
x |
140 |
x |
|
253 |
|
|
|
|
|
|
|
|
x |
x |
x |
x |
|
262 |
|
x |
120 |
x |
x |
|
263 |
|
100 |
x |
x |
x |
|
270 |
|
x |
x |
x |
175 |
|
285 |
|
x |
x |
x |
x |
|
306 |
|
|
|
|
|
|
|
|
x |
140 |
170 |
x |
|
307 |
|
x |
x |
x |
200 |
|
325 |
|
x |
x |
x |
x |
|
349 |
|
x |
160 |
x |
x |
|
351 |
|
130 |
x |
x |
x |
|
352 |
|
|
|
|
|
|
|
|
|
180 |
x |
x |
|
395 |
|
|
x |
220 |
x |
|
398 |
|
|
x |
x |
250 |
|
407 |
|
|
200 |
x |
|
|
438 |
Sources: Adapted from Saltman (1991) and
Scott (1989).
Table 8-3. Factors for converting
basis weights from one basic size to another.
Convert to:
|
g/m2
|
17 x 22-500
|
24 x 36-500
|
25 x 38-500
|
25 x 40-500
|
1,000 ft2
|
Actual ft2
|
|
|
|
|
|
|
|
|
|
Convert from:
|
1.000
|
0.266
|
0.614
|
0.676
|
0.711
|
0.205
|
-
|
g/m2
|
|
|
|
|
|
|
|
17 x 22 - 500
|
3.759
|
1.000
|
2.311
|
2.541
|
2.675
|
0.770
|
1,300
|
24 x 36 - 500
|
1.627
|
0.451
|
1.000
|
1.100
|
1.158
|
0.333
|
3,000
|
25 x 38 - 500
|
1.480
|
0.394
|
0.909
|
1.000
|
1.052
|
0.303
|
3,300
|
25 x 40 - 500
|
1.406
|
0.374
|
0.864
|
0.950
|
1.000
|
0.288
|
3,470
|
1,000 ft2
|
4.882
|
1.299
|
3.000
|
3.298
|
3.472
|
1.000
|
1,000
|
|
|
|
|
|
|
|
|
|
|
|
|
|
|
|
|
|
|
Source: Scott (1989).
Example: To convert from 60 lb of size (25 x 40-500) to weight
of basic size (17 x 22-500) look up the multiplying factor associated
with (25 x 40-500) in the "Convert from" column and (17
x 22-500) in the "Convert to" row. The new basis weight
is calculated as follows:
60 lb
(25 x 40-500) x 0.374 = 22.4 lb (17 x 22-500).
Table 8-4. Representative
thickness values of several grades of paper and board.
|
Paper
|
mm
|
Inches
|
"Points"
|
Micrometers
|
|
|
|
Capacitor |
0.0076 |
0.0003 |
0.3 |
7.6 |
|
|
Glassine |
0.03 |
0.0012 |
1.2 |
30 |
|
|
Facial tissue |
0.065 |
0.0025 |
2.5 |
65 |
|
|
Tablet |
0.075 |
0.003 |
3.0 |
75 |
|
|
Newsprint |
0.085 |
0.0033 |
3.3 |
85 |
|
|
Duplicator |
0.095 |
0.0037 |
3.7 |
95 |
|
|
Rag bond |
0.11 |
0.0043 |
4.3 |
110 |
|
|
Kraft envelope |
0.13 |
0.0051 |
5.1 |
130 |
|
|
Index bristol |
0.25 |
0.0098 |
9.8 |
250 |
|
|
Corrugating medium |
0.23 |
0.009 |
9.0 |
230 |
|
|
Linerboard |
0.23-0.64 |
0.009-0.025 |
9-25 |
230-640 |
|
|
Chipboard |
0.15-0.41 |
0.006-0.016 |
6-16 |
150-410 |
|
|
Cardboard blanks |
0.30-2.0 |
0.012-0.0780 |
12-78 |
300-2,000 |
|
|
Source: Scott (1989).
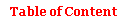
|